Magnetic recording on nanostructures
Present magnetic disk drives are based on perpendicular recording systems where the magnetization of the recorded bit points perpendicular to the disc plane. These systems contain a recording head composed of a separate read and write element, which flies in close proximity of about 15 nm to a granular recording medium.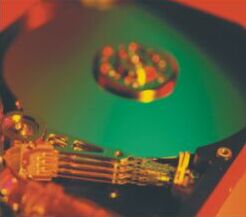
The inductive write element records the data in vertical magnetization patterns. The information is then read back with the giant magnetoresistive (GMR) or tunneling magnetoresistive (TMR) read element by measuring the stray magnetic field from the transitions between regions of opposite magnetization. Finally, a signal processing unit transforms the analog readback signal into a stream of data bits.
The fine microstructure of the granualar recording media as shown in the TEM-image below allows writing and storing
transitions at almost any location and at high linear densities with the ultimate density
limited by the grain size. As illustrated in the inset of the TEM-image
the transitions follow the grain boundaries, which provide strong pinning sites for
the magnetic transitions. Finally, a thin carbon overcoat and a lubricant layer protect
the media from oxidation and physical damage as a result of physical contact with the head.
To increase data-storage densities while maintaining acceptable performance, designers have shrunk the media's grain diameters and decreased the thickness of the media as well as the physical dimensions of the read/write heads. The resulting smaller grain volume V makes them increasingly susceptible to thermal fluctuations, which decreases the signal sensed by the drive's read/write head. If the signal reduction is great enough, data could be lost in time due to this superparamagnetic effect.
The following simple model illustrates this thermal effect. The weak intergranular exchange coupling between grains allows the perpendicular recording medium to be approximated as a collection of independent particles. The energy barrier for magnetization reversal in the presence of an external magnetic field H is given by EB(H, V) = KuV (1 -H/H0)n, (1)
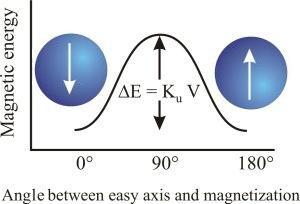
Theoretical and experimental analyses arrive at the thermal stability condition KuV > 55kBT to maintain sufficient signal stability over at least five years of data storage. This criterion sets an effective limit on the minimum grain volume and was the basis for the predictions that areal densities would be limited by thermal activation of the grains. In the presence of these thermal effects, a variety of material and system changes can be implemented to lessen their impact. Thermal stability is improved by reduction of crystallographic defects, by improving epitaxy, and by materials with higher anisotropy for the recording media, where the increase in Ku counters the reduction in V to maintain the thermal stability.
The latter is currently achieved by increasing the Pt content in modern CoPtCr-SiO2 media. Other media candidates with high anisotropy are the tetragonal L10 phases of FePt and CoPt, artificially multilayered materials and rare earths compounds. Nevertheless, following this path also increases the write field requirements, since the write field Hw ~ Ku / Ms, where Ms is the saturation magnetization of the recording media. The required write field improvements were traditionally achieved by design changes in the write head and the use of materials with higher saturation magnetization as the write poles. However, modern write poles already consist of low-anisotropy materials with saturation magnetization density approaching the highest reported value, which will limit the maximum achievable write field.
It is becoming increasingly clear that, to avoid limitations to areal growth rates arising from thermal instabilities in future high-areal-density products, significant improvements of the recording medium must be achieved. New thin film materials or architectures are needed that ease the write field requirements at high areal densities and are more thermally stable than conventional media. These new concepts include:
Patterned media
The creation of lithographic magnetic nanostructures or “patterned media” for single-bit
data storage is recognized as a promising approach to overcome the areal density limit for
magnetic recording imposed by superparamagnetism in conventional magnetic media.
As an approach to extend magnetic storage densities we want to investigate
patterns of magnetic nanostructures with perpendicular magnetic anisotropy. Each
nanostructure can be used for storing a single bit of information. Being single domain,
the bit size can be reduced compared to conventional storage media without
losing thermal stability.
BPM implies a formation of an ordered at a large scale 2D-array of magnetic nanostructures with a size of about 10 nm and period below 25 nm.
Hard magnetic alloys (i.e. FePt) have to be used to satisfy the criteria for long time stability of the stored information bit [1].
However, reduction of a size of nanostructures below certain critical one results in a modification of the magnetization reversal mechanism from nucleation
and domain wall propagation (small coercive field) to coherent rotation of magnetization in a single-domain nanostructure.
In the latter case, the coercive field required to reverse the magnetization of a bit is equal to anisotropy field (~11 T for a single-domain FePt nanostructure).
This field is substantially larger compared to the write field provided by a recording head (< 2 T) [2]. This discrepancy is known as a writeability issue.
There are two approaches suggested to reduce the switching field of the magnetic nanostructure: (i) heat assisted magnetic recording (HAMR) and (ii) exchange
coupled composite (ECC) media. HAMR relies on the local heating of the magnetic nanostructure close to the Curie temperature before writing event [3]. In contrast,
magnetic bit in the ECC media concept [4, 5] consists of two magnetic components: soft and hard parts. In this composite, the thermal stability is given by the
hard component, while the switching field gets substantially reduced due to the early nucleation of the reverse domain in the soft layer resulting in a torque
at the interface between hard and soft layer influencing the nucleation field of the hard layer. Both approaches have potential for implementation
in the future generation of magnetic storage devices.
![]() Fig. 1: Perpendicular magnetic nanostructure arrays (period 100nm). |
![]() Fig. 2: Magnetic images of self-assembled particle arrays after Co/Pd film deposition (particle size 310 nm). |
![]() Fig. 3: Schematic of the magnetic recording process on patterned perpendicular media using a conventional longitudinal recording head. |
![]() Fig. 4: Top: AFM image showing a CoCrPt island row with a period of 103 nm which has been patterned by FIB lithography. Bottom: Corresponding MFM image after in-phase writing revealing alternating magnetization orientations of the written islands. (b) MFM image taken after sequentially writing of four equidistant islands in a dense island array (period: 103 nm). |
Percolated media
Percolated media represent a very promising solution to address the thermal stability issue. In such a medium, the thermal stability is determined by the pinning field of domain walls on randomly placed defects. The pinning sites can be non-magnetic or soft magnetic defects with a diameter of 2 nm, in order to get a sufficiently small transition jitter. In our approach, we apply an organic/inorganic self-assembly approach using block copolymer micelles to create an array of nanoperforations. This pattern is used as a template for the deposition of Co/Pt multilayers with out-of-plane magnetic anisotropy. The magnetic hysteresis loop of this system show two distinct parts, which are related to the reversal of the continuous film and the array of magnetic nanodots created in the nanoperforation. Strong pinning of magnetic domain walls at the nanoperforation locations was observed (see Fig. 5). Therefore, this approach of easy pattern formation along with magnetic film deposition is considered to be promising to create a percolated magnetic media, which is attractive for future generation of magnetic storage devices. However, a further reduction of the pattern period as well as the diameter of nanoperforations combined with a magnetic film with stronger out-of-plane magnetic anisotropy is required.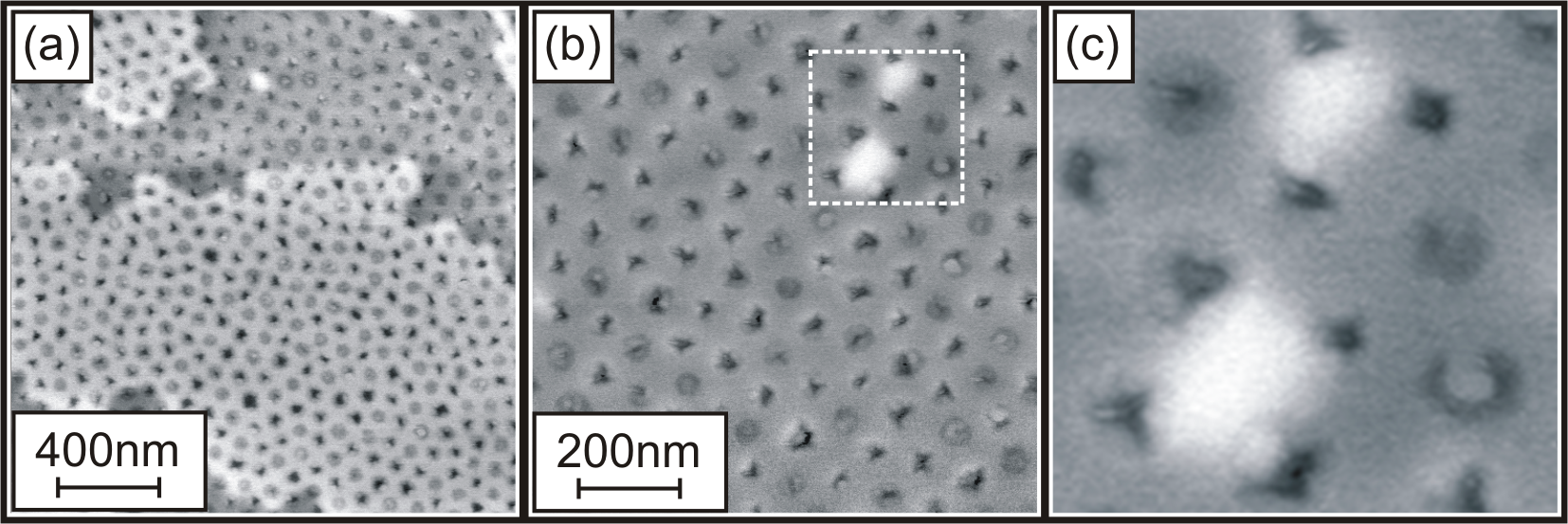
Fig. 7 (a), (b): Superimposed AFM/MFM images. The MFM images were taken in remanence after applying a reverse field. (c) Enlarged image of the marked region in (b).
[1] D. Weller and A. Moser, IEEE Trans. Magn. 35 (1999) 4423.
[2] S. Batra, J. Hannay, H. Zhou, and J. Goldberg, IEEE Trans. Magn. 40 (2004) 319.
[3] W. A. Challener, Chubing Peng, A. V. Itagi, D. Karns, Wei Peng, Yingguo Peng, XiaoMin Yang, Xiaobin Zhu, N. J. Gokemeijer, Y.-T. Hsia, G. Ju, Robert E. Rottmayer, Michael A. Seigler, and E. C. Gage, Nature Photonics 3 (2009) 220.
[4] D. Suess, T. Schrefl, S. Fähler, M. Kirschner, G. Hrkac, F. Dorfbauer, and J. Fidler, Appl. Phys. Lett. 87 (2005) 012504.
[5] D. Suess, J. Magn. Magn. Mater. 308 (2007) 183.
[6] D. Makarov, J. Lee, C. Brombacher, C. Schubert, M. Fuger, D. Suess, J. Fidler, and M. Albrecht, Appl. Phys. Lett., 96 (2010) 062501.